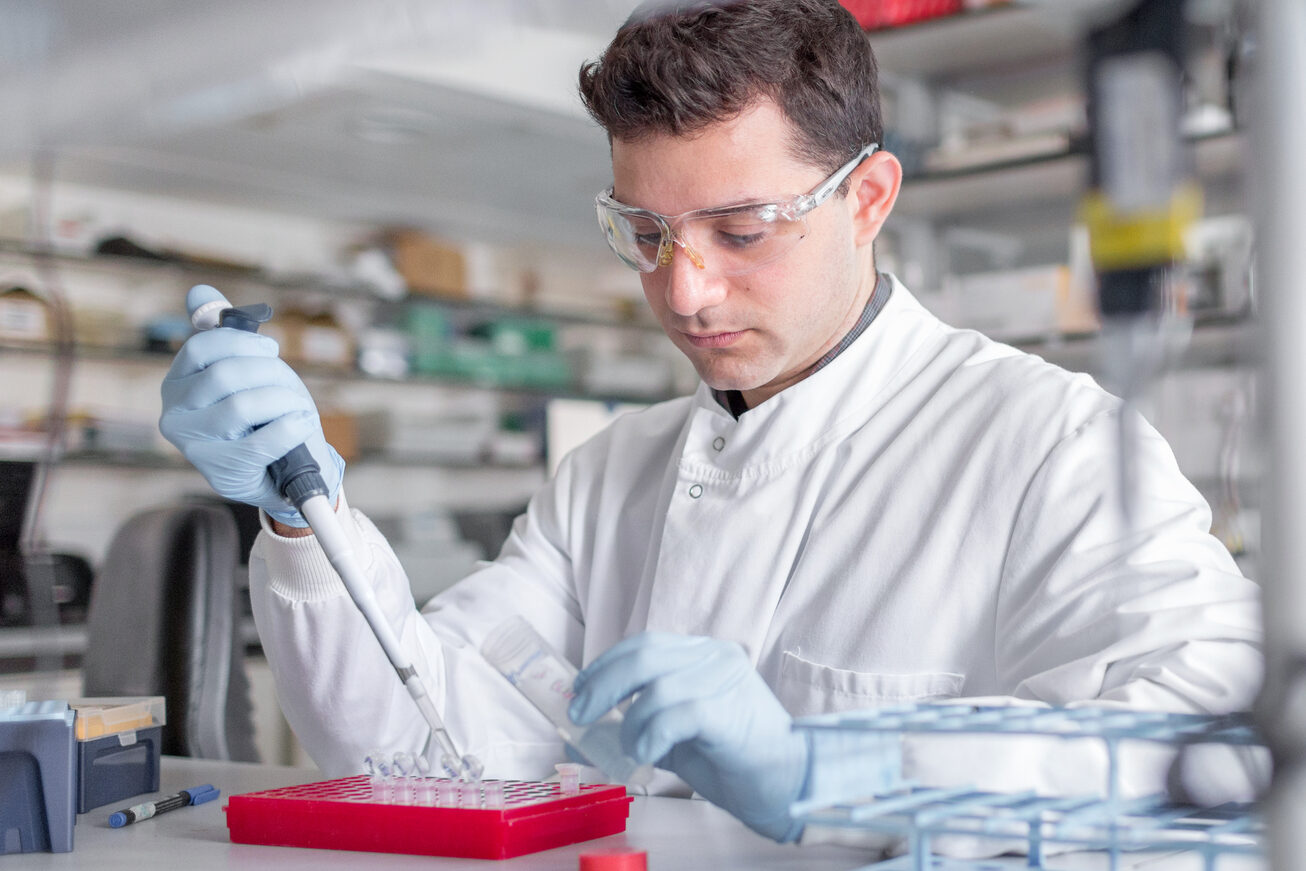
By producing cells outside the body, researchers from Imperial’s Faculty of Medicine have been able to replace animal procedures while delivering better scientific results.
Researchers are often limited in the work they can do with humans by how hard it is to reach certain organs. The brain and the heart are challenging to work on, and tissues deep in the lungs or intestine are almost impossible to reach in living subjects. This is one reason they turn to animal models. At Imperial, however, several groups are building tissues outside the body, making them easier to study. This often produces better scientific results, while reducing or replacing animal procedures.
Several of these approaches involve using stem cells, the cells responsible for building or repairing tissues in the body. Some of these stem cells, known as pluripotent stem cells, can take on a wide range of specialised functions, depending on the signals they get from the body.
Researchers are now able to produce pluripotent stem cells from ordinary body cells, such as skin cells, tooth pulp or blood cells. They can then reprogramme them and grow them in cell culture ‘lines’ that develop into the tissues they want to examine.
From the heart
Professor Sian Harding and her group at the National Heart and Lung Institute have used this technique to produce cardiomyocytes, the cells in the heart responsible for the organ’s rhythmic beating. And just like heart muscle, these cells pulse spontaneously
when cultured in a dish. “After about ten days they start to produce this beating effect,” says Professor Harding.
The cells can also be made into heart tissue, and when a small strip is attached to two silicon posts, it pulls rhythmically at the supports, essentially working out. Measuring these contractions, or looking at fluctuations in calcium within the tissue culture, can be used to answer a range of research questions.
The advantage is not only that this heart tissue is human, but if the stem cells are produced from patients with heart conditions, these appear in the cultured tissue as well. “If people have the mutations that cause poor contraction or rhythm disturbances, in many cases we’ve been able to show that you can see these conditions either in the cells or in the engineered heart tissue,” explains Professor Harding.
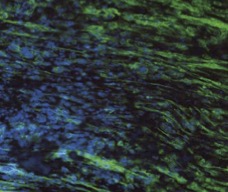
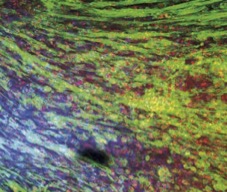
“You can then investigate that mutation, in a human background, without having to produce transgenic mice. With new gene editing technology you can put the mutation into a normal line or take it out of that line, and so you can understand what is happening in the background and what is happening due to the mutation. And you can test drugs on it.”
The method can also be used as a more general screen to see if drugs for other conditions have an adverse aect on the heart. This is o!en the case if they aect the hERG channel, a structure that contributes to the electrical activity of the heart and helps coordinate beating.
“A lot of drugs are thrown out of development because they have hERG channel activity, and you can see the electrical potentials changing in the cardiomyocytes,” says Professor Harding.
A response to dementia
Dr David Owen in the Department of Medicine is also working with material developed from pluripotent stem cells, to study the role of the immune system in dementias such as Alzheimer’s disease.
“The immune system in the brain is probably not the underlying cause of these diseases, but it may be making things a lot worse,” he explains. For example, it may become less effective at cleaning up the damage caused by dementia, or start to damage the brain as the dementia progresses.
Investigating this in the living human brain is practically impossible, and animals often provide poor models. For example, stimulating the immune response in a mouse’s brain will increase production of translocator protein (TSPO), the molecule Dr Owen is studying, while levels fall when the human brain is stimulated.
So he and his colleagues took pluripotent stem cells, derived from human skin, and turned them into cells that resemble microglia, which are responsible for the immune response in the brain. “That enables us to study human cells, and get around all the problems of species differences.”
As new information about the immune response emerges from these human microglia-like cells, it can be followed up in other human settings. One option is to look for the same phenomena in macrophages, the immune cells in the blood, with experiments involving volunteers or patients.
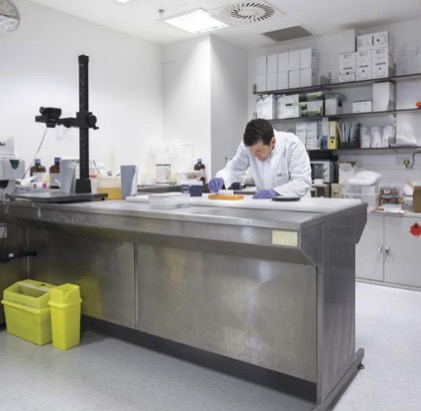
“If we want to find a cure, something we are still some way from doing, we really need to understand pancreatic islet biology”.
– Dr David Owen, Department of Medicine
Another is to cross-check the findings with post-mortem samples, such as those held in the Multiple Sclerosis and Parkinson’s Tissue Bank at Imperial, or to run brain imaging experiments on patients. “You make a discovery in a cell line, but to see whether or not it is relevant you need to look directly at the brain,” says Dr Owen.
He is particularly keen on imaging, for its scientific potential and as a way of avoiding animal procedures. “Imaging in humans was not developed as a means of reducing animal experimentation, but it is a very nice side effect.”
Out of the lungs
The difficulty in creating accurate animal models of the human immune response is also behind the work of
Mr Tankut Guney, currently completing a PhD in the National Heart and Lung Institute. He is interested in lung diseases, such as asthma and chronic obstructive pulmonary disease (COPD), in which immune response plays an important role.
“We don’t have very representative animal models for the lung,” he explains. “The mouse immune system is different from that in humans, and the way lung repair happens in mice is also completely different, which is why they don’t get asthma or COPD.” Underlining these differences, drugs developed with animal models often fail when they reach clinical trials.
So Mr Guney has been working with organoids, small three-dimensional replicas of lung tissue derived from human cells. Rather than using pluripotent stem cells, he starts with the progenitor cells whose role is to repair the inner surface of the lung in case of damage.
Grown outside the body on a basement membrane, these progenitor cells independently form spheres, which in turn develop a cavity or lumen in the centre. “On the outside of the sphere you have an undierentiated stem cell population that regenerates the inside, and inside you have functional cells, such as mucus producing cells or cells with beating cilia,” Mr Guney says.
Working on these organoids not only gets around the differences between humans and other animals, but allows research on individuals with different medical histories. For instance, Mr Guney is currently comparing responses to cigarette smoke in organoids drawn from people who have never smoked and those who have COPD.
This promises to increase knowledge about the factors that lead to COPD and help identify potential treatments. “It may not give us the complete answer, but it will give a good indication of where to go, without having to use a lot of mice in the process and then risk a high percentage of those treatments failing in clinical trials.”
Building small guts
While most eeffective when using human tissues to replace animals, the notion of building tissues outside the body can also help reduce the use of animals. Professor Irene Miguel-Aliaga, of the Institute of Clinical Sciences, is doing this in her work on how the intestine adapts to challenges such as changing diet or pregnancy.
“There is increasing evidence that these intestinal adaptations are key to maintaining our normal physiology,” she says. “It is also increasingly recognised that, when they fail, they contribute to obesity and diabetes.”
Complementary in vivo and in vitro approaches have been developed to explore how these adaptations take place. “We first characterise them in fruit flies (which have complex and adaptable intestines too) and then explore their broad relevance in intestinal organoids derived from mice. These are miniature versions of the mouse gut which you can grow – and genetically manipulate – on a dish.”
Rather than embryonic stem cells, these organoids are produced from stem cells dissociated from the adult mouse’s small intestine. But only a small number of animals are involved.
“You can derive many organoids from one single mouse and you can maintain them on a dish for months, even years,” says Professor Miguel-Aliaga. “This reduces the need for continuously breeding mice and also reduces the overall number of mice involved.”
She is enthusiastic about the potential for taking this approach further. “Organoid technology is in its early days and the more we can learn about the organoids themselves, the easier it will be to determine whether certain genes affect their structure and/or physiology.”
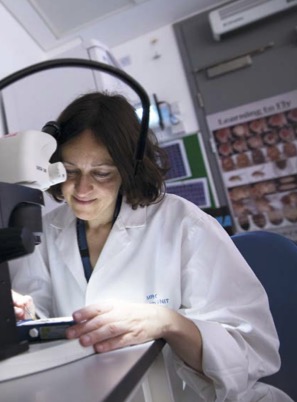