Imperial college researchers always think about Relevance when using animal models for they study. This fourth R means ensuring that research with animals relates to human disease and will ultimately have an impact on human health.
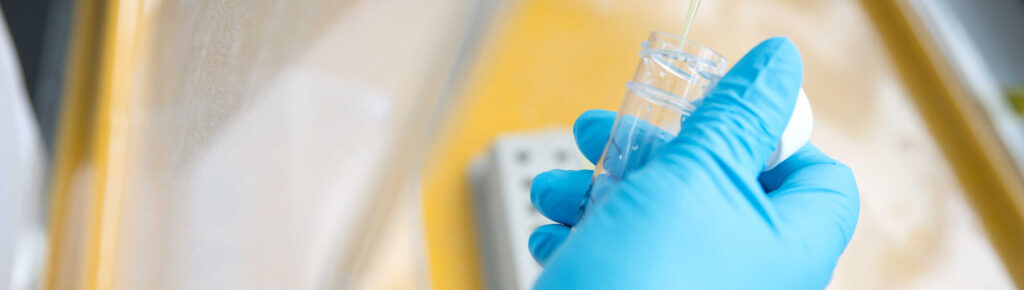
The long game in obesity research
The relevance of animal research is exemplified by work on conditions such as obesity. “If you are looking at food intake, you can’t measure it in anything other than the whole animal,” says Dr James Gardiner, Reader in Molecular Physiology in the Faculty of Medicine. “You are looking at an interaction between multiple systems, and the animal has to move to get food, which is a complex behaviour.”
Even the release of insulin, which regulates the level of blood sugar after feeding, demands a whole-animal approach. “There is an interaction between the brain, the pancreas and the gastro-intestinal tract. All of those come together, and currently there is no model system that allows us to look at all of those things in vitro.”
Imperial’s research on obesity has had significant impact, even if it takes decades to reach the clinic. It was in 1996 that its researchers, led by Professor Sir Stephen Bloom, first demonstrated that the hormone glucagon-like peptide 1 (GLP-1) plays
a role in regulating eating. Subsequent work with rats showed that introducing GLP-1 directly into the brain, or blocking its effect, could influence feeding behaviour, demonstrating a physiological connection.
But GLP-1 also stimulates the release of insulin after eating, which led to it being investigated as a possible treatment for type 2 diabetes. This condition, in which insufficient insulin is produced by the body, is closely associated with obesity. GLP-1 and its analogues were found to help both insulin production and appetite, and as a result they are now widely used as a therapy.
“This is one of the few treatments that can be used in type 2 diabetes that leads to weight loss rather than weight gain,” says Dr Gardiner. Meanwhile one of the analogues has recently been approved in the USA as a treatment for obesity before diabetes sets in. “You are catching the problem before it develops.” The 3Rs played an important part in the work that led to these new therapies,
in particular because animal welfare has an influence on appetite and feeding.
“ The aim of everything we do is to improve treatment.”
– Dr James Gardiner, Department of Medicine
“If you have an animal that is stressed, it won’t respond, so you have to ensure that the animal is not stressed, is not ill in any way, and is not suffering.” While the GLP-1 research has produced successful new therapies, this does not mean that the research is over. “In each individual person there will be differences, and while some respond well to treatments, others won’t.”
Dr Gardiner’s most recent work focuses on thyroid hormones. These play a role in controlling appetite, but have many other functions in the body, so any drug mimicking these hormones or interfering with the receptors through which they work must be very precisely targeted if it is not to have severe side effects.
As a first step, Dr Gardiner and his colleagues succeeded in genetically modifying mice so that a particular kind of thyroid hormone receptor in their brains no longer worked. The mice were healthy, but very hungry. “When given free access to food, they would just eat and eat. We ended up with very fat mice.”
This in turn opens the way for more precisely targeted drugs, although as with GLP-1 this research may take decades to come to fruition. “The work that we are doing currently will take time to be translated to the clinic, but then the aim of everything we do is to improve treatment. No-one is really interested in fat mice, they are interested in treating obesity.”
Unravelling the fundamentals of cell development
Imperial’s emphasis on impact does not mean that basic, curiosity-driven research is side-lined. On the contrary, some of the best examples of relevance deal with attempts to unlock the basic mechanisms of human biology.
Take the work of Professor Richard Festenstein, who is based in Imperial’s Brain Sciences Division. He is interested in how genes are turned on and off during the development of different types of cells, and how these decisions are ‘remembered’ when cells divide.
Active genes are found in stretches of the chromosome with a loose, open structure, known as euchromatin. This contrasts with tightly packed areas of the chromosome, known as heterochromatin, which do not contain active genes.
Studies in fruit flies showed some time ago that when a gene is placed closer than usual to a region of heterochromatin, this increases the probability that it will be switched off. This is known as position effect variegation. However, it was not until 1996 that Professor Festenstein and his colleagues were able to show that a similar phenomenon could be found in mammals.
The next step was to look at how this gene silencing effect might be taking place. “There are at least 100 modifiers of the extent of this gene’s silencing, and those modifiers turned out to be the molecular components of heterochromatin. So, the actual mechanism of silencing was worked out by looking at these modifiers.”
These questions about the fundamentals of gene control also had a practical goal in mind. One of the characteristics of heterochromatin is that it contains repetitive DNA sequences, in particular triplets that repeat over and over again. Such repeats are also seen in abnormally high levels in a number of incurable human genetic diseases. “We went on to see whether repeats that expand in human diseases could also induce this kind of gene silencing,” says Professor Festenstein.
This was done by producing transgenic mice with varying numbers of triplet repeats in the heterochromatin. These were linked with a gene whose activity could be easily detected – a ‘reporter gene’ – that would show that silencing was taking place, without creating any disease or discomfort in the animals.
“We found that the repeats induced a very similar type of silencing to that seen in humans, and this was also sensitive to the genetic modifiers that modified position effect variegation.”
Connecting triplet repeats in heterochromatin with gene silencing opened up the possibility that targeting heterochromatin might be a way of turning silenced genes back on again. The test case was Friedreich’s ataxia, a degenerative disease in which nerves controlling movement are progressively damaged.
This is a rare disease, affecting 1 in 30,000 people, for which there is no existing therapy. It normally occurs in childhood, with individuals developing clumsiness, slurred speech, and impaired hearing. An associated form of heart disease is frequently fatal.
“We went on to work on a mouse model for Friedreich’s ataxia, as well as looking in patients’ cells at various known inhibitors of the enzymes that are involved in the silencing mechanism,” Professor Festenstein explains.
“We are now preparing to do a clinical trial to see whether this is an effective therapy.”
– Professor Richard Festenstein, Department of Medicine
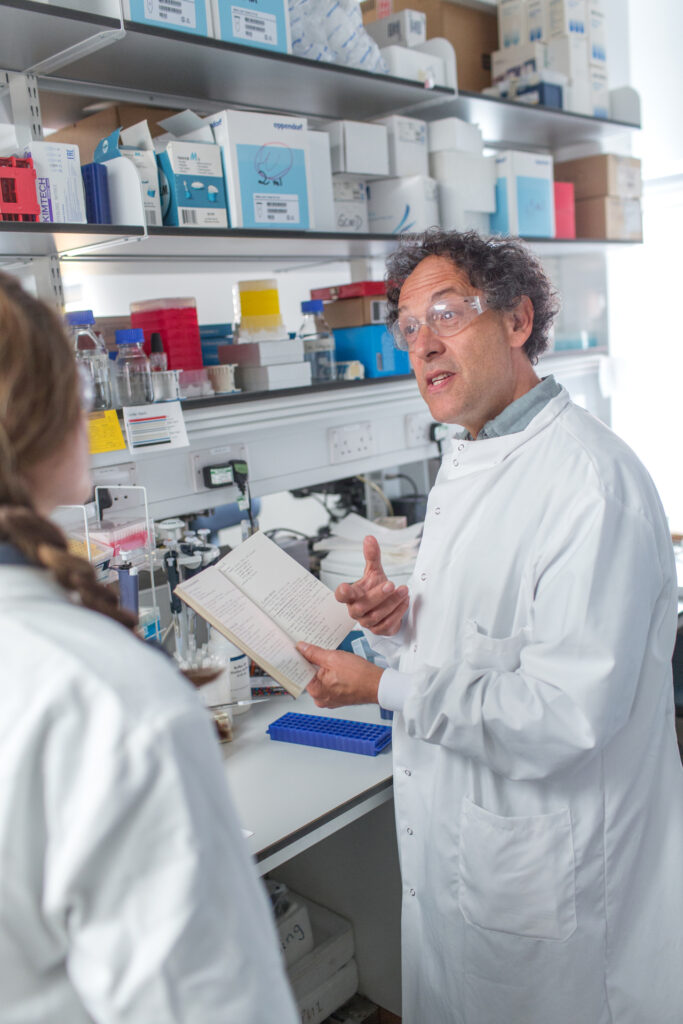
They found that high doses of vitamin B3 could switch the gene back on, by modifying the heterochromatin. “In mice, and in cells from people, we have shown that we can restore expression of the gene that is aberrantly silenced in the disease. So this is potentially a radical therapy for this disease.”
This result was reached without subjecting mice to the full-blown disease. Even with a mild form, it was clear when the gene had been turned back on. A subsequent study with Friedreich’s ataxia patients showed that high doses of vitamin B3 were able to turn on the gene that is turned off in the condition. “So, we are now preparing to do a clinical trial to see whether this is an effective therapy.”
Professor Festenstein thinks that Friedreich’s ataxia is just the beginning. “We see this as a potential proof of principle, that other diseases caused by a similar mechanism might also be amenable to this kind of treatment,” he says. “It has taken quite a while to get to this point, but it is looking very promising in terms of being able to break into disease areas that previously have been completely intractable to therapies. And the use of the mice was crucial in this process.”